GREEN PAVEMENT WITH HIGH VOLUME FLY ASH CONCRETE
Anil Banchhor
Chief Executive (Concrete Business), ACC Limited
Abstract:
This paper discusses the suitability of HVFAC in road construction on the basis of a demonstration project wherein a 30m-long road was constructed with an embedment of 63 nos vibrating wire type strain gauges to measure the temperature and load stresses under actual load conditions. Panels of different lengths, thickness, with/without polythene sheet between DLC & PQC, etc., were cast for evaluation. The benefits of using fly ash in concrete pavements have been evaluated in the above demonstration project in terms of fresh concrete properties and hardened concrete properties with respect to strength and durability. With the improved properties of HVFAC, the initial cost also comes down because of reduced pavement thickness and the cost difference with the bitumen road also getting reduced coupled with the improved life cycle cost.
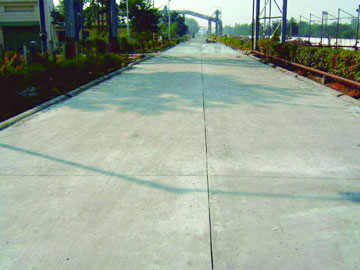 |
The design of concrete pavement in India is being done as per the standard code of IRC-58–2002, wherein load stresses as well as temperature stresses are predominant and have a cumulative effect due to extreme heat in the summer. The design is based on the empirical formulae of Bradbury’s coefficient and Westergaard’s equations. With this background, an experimental study project was taken up at Noida to study the effect of temperature and load stresses in the HVFA concrete panels and to compare the stresses with that of control concrete made with similar materials and workmanship at the same locations by measuring the actual stresses by inserting the strain gauges (vibrating wire type) at various depths and locations of concrete pavement. The author concludes that the use of PPC would also solve the problem of non-availability of facilities of testing of fly ash at many construction sites, where the processed fly ash as per IS 3812-2003 is not available but the user wants to derive the benefits of good quality processed fly ash present in the cement.
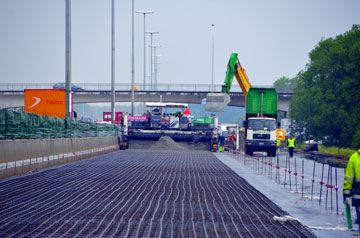 |
INTRODUCTION
In India, cement concrete roads have been built in isolated locations on national highways, state highways and city roads since early 1950. In the last decade of the 20th century, a few major road projects like the Mumbai–Pune expressway (90 km), the Delhi–Mathura (100 km section) highway and the Indore bypass road (35 km) were undertaken with concrete pavement. These experiences accelerated the construction of 1600 km concrete road in the Golden Quadrilateral. Further, the advantages of cement concrete road over bitumen road in terms of longer life, less maintenance, good riding quality, better skid resistance, lower life-cycle cost, free of effects of rain water and spillage of oil, better reflectivity, saving in fuel consumption, etc., have also led to the construction of more and more concrete roads in several parts of India.
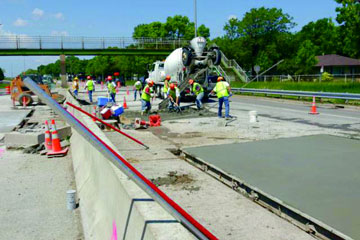 |
However, due to the higher initial cost and the requirement of a higher degree of care in design and construction, the concrete roads were not preferred to bitumen roads. Now that major road projects are being implemented using modern equipment, there has been substantial confidence and capacity building among the engineers and the contractors in the area of concrete roads. The cost difference between concrete and bitumen roads, which used to be over 50% earlier, has come down to 15 to 25% now. This gap has further come down to 10 to 15% due to the adaptation of high volume fly ash technology in concrete roads.
High volume fly ash (HVFA) concrete was developed nearly two decades ago. In this type of concrete, about 40-60% of portland cement is replaced by a suitable quality of fly ash for different applications, including concrete pavements. HVFA concrete exhibits improved fresh concrete properties,
excellent mechanical properties and long-term durability along with economy.
An improvement in the properties of HVFA concrete with respect to bleeding, setting time, autogenous temperature rise, development of compressive strength with age, flexural- and splitting- tensile strengths, drying shrinkage and resistance to abrasion along with the efficient use of cementitious material is leading to the increased use of HVFA concrete in various applications in concrete constructions.
This paper shows that good quality HVFA concrete can be produced with the judicious use of cement and superplasticisers for different structural applications with varying percentage of fly ash additions. It can be used successfully in our country with very low life-cycle cost and good performance, because fly ash is abundantly available and the temperature is also conducive for better reactivity of fly ash as compared to countries like Canada and the US, where the cold climate prevails for most part of the year.
HIGH VOLUME FLY ASH CONCRETE
High volume fly ash concrete typically should have cement replacement levels of about 40-60% by suitable quality fly ash. However at this level of replacement, there is a drop in the early age strength of concrete, which would in turn slow down the speed of construction activities, if proper precautions are not taken. For many structures like roads, raft foundations and mass concrete members, this may be acceptable, but for normal construction, there is still an apprehension in the minds of users of normal concrete.
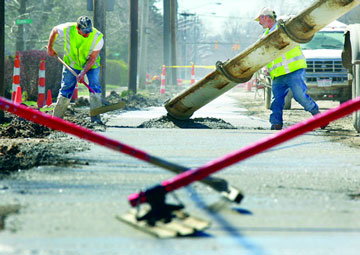 |
According to Malhotra [10], high-performance, high-volume fly ash concrete with an early strength of 6–8 M Pa on 24 hrs and 28 days strength of 40Mpa would be possible at an ambient temperature of 40 degree centigrade with a water content of 120–135 kg and cement factor of 180–200 kg per cubic metre. This concrete would require the use of a superplasticiser dose of 2 to 3 kg per cu. m. to get the desired slump and slump retention. The water to cementitious ratio should be less than 0.32 to 0.35, and the fineness of the fly ash needs to be in the range of 250–350 sq. m. per kg. Very fine fly ash will increase the water demand and any increase in quantity of water would lead to drop in strength.
This technology is particularly suitable for the Indian climate, where the average ambient temperature ranges from 32 to 42 degree centigrade for most part of the year. In cold countries, like Canada and the US, this technology has successfully been used for conventional concrete structures like RCC buildings, water tanks and bridges. Some examples are the Liu Station, the Parklane project, the BC gas building, the Brentwood Station and the Bayview high-rise buildings.
In our country, several demonstration projects for roads have been successfully completed. The first high volume fly ash building in India using up to 50% fly ash was constructed in 2005 in Amethi (UP). Many high-rise buildings in Mumbai use concrete with fly ash replacements up to 30% under strict quality control. This is an encouraging trend for the use of HVFA concrete, which certainly has a great future in India.
CONSIDERATIONS ADOPTED FOR MIX DESIGN OF HVFA CONCRETE
The following important considerations are adopted for mix design of HVFA concrete:
- The water content is kept between 120–135 kg.
- Cement content of 180-225 kg per cubic metre is generally sufficient for M-40 grade concrete.
- The water to cementitious ratio is kept between 0.32 and 0.35
- A superplasticiser dose of 2 to 4 kg per cu. m. is required for low water content concrete to make the mix workable.
- The fineness of the fly ash needs to be in the range of 320–350 sq. m. per kg. Very fine fly ash would increase the water demand and require PCE-based admixtures. Retention on 45-micron sieve should not exceed 34% and preferably be less than 15% for good pozzolanic reaction. The fly ash should conform to IS 3812-2003. The loss of ignition should be less than 5%; otherwise, there will be loss of slump due to absorption of superplasticiser on the carbon particles.
- Coarse aggregates were well graded and of igneous origin, like granite and basalt. Sand pertaining to zone-1 with silt content less than 1% was used.
PROPERTIES OF HIGH VOLUME FLY ASH CONCRETE OBSERVED
- Workability and the slump The dosage of plasticiser may vary, depending upon the characteristics of cement and fly ash used and their proportion. Hence, a compatibility test was done to choose the best performing combination before mix design. Fly ash makes the concrete cohesive but sticky, and the flow of the mix is reduced at low water content. However, on vibration, the mix flows very well and compactibility and finishability is enhanced. The slump of the concrete required for roadwork varies from 10 to 40mm at the point of pour, and HVFA concrete flows smoothly under vibration.
- Bleeding and setting time The bleeding ranges from very low to negligible due to low water content. Therefore, proper precautions should be taken to cover the concrete with plastic sheet or moist hessian cloths immediately after finishing/texturing of the concrete surface and early curing by sprinkling of water should be started. In OPC concrete, the movement of bleed water creates channels and forms a weak interfacial zone along the aggregates surface and microcracks develop in this region. In case of HVFAC, the bleeding is very low, which results in a stronger transition zone. The setting time of HVFAC is longer as compared to OPC concrete due to low cement content, but the striping time is observed to be same.
- Autogenous temperature Because of low cement content, the autogenous temperature rise is very slow. Hence, the chances of thermal cracking, common in OPC concrete, are very much reduced. The thermal gradient between the surface and the interior of the concrete is less than 20o C, which prevents thermal cracking.
- Joint cutting The normal joint cutting for OPC concrete is 16 to 24 hours. However, in the case of HVFA concrete, it can be delayed by 48 hours since the hardening is slow. This gives an added flexibility to the cutting. The cut made in HVFA concrete had sharp edges as in OPC concrete.
- Curing Like other types of concrete, HVFA concrete also needs to be adequately cured. Early curing is a must to prevent plastic shrinkage cracks in hot and windy weather. To attain higher long-term strength, low permeability and long-term resistance to aggressive agents, etc., curing for 10–14 days is adequate.
- Compressive and flexural strength One-day strength of 8 to 10 Mpa is achieved with HVFA concrete, which is sufficient for de- shuttering of forms. The compressive strength keeps on increasing even up to 180 days and beyond due to pozzolanic activities and the pore-filling effect. Higher compressive strength imparts higher flexural strength and higher modulus of elasticity and shear resistance. The improved interface transition zone between the paste and the aggregate reduces microcracking. The flexural strength of HVFAC also increases over a period of time, which helps resist bending. One important finding of the study is that the ratio of flexural strength to compressive strength is higher in the case of HVFA concrete as compared to control concrete.
- Water permeability The water permeability of HVFA concrete is found to be very low in the order of 20 mm, due to better pore-filling effect. This property helps in resisting the penetration of water, sulphates, chlorides, etc., prevents the corrosion of embedded tie bars and dowel bars and resists alkali silica reaction.
- Drying shrinkage Drying shrinkage cracks have been reported in OPC concrete. The problem is normally noticed after 3 or 4 months. This distress is related to the drying of cement paste and occurs when the cement paste is more due to higher cement and water content. In HVFAC, due to control on water and cement content, the paste volume is found to be lower by 25 % and the drying shrinkage strain lower by 10 % when compared to OPC concrete. The measured value on a 50% fly ash HVFAC is 372X10-6 on 142 days (ASTM C 490).
- Abrasion Resistance The water to binder ratio plays an important role along with the use of well-graded, hard granite aggregates, good compaction with needle or screed vibrator, protection of concrete from sun and wind, curing for a minimum of fourteen days, etc., which are requirements for very good abrasion resistant concrete. In HVFA concrete, the loss due to abrasion on a test as per ASTM 779C varied only between 1.2 mm and 1.8 mm over 20 minutes on 90 days, which was well within the recommended limit of 2mm for good concrete.
THE PROJECT
The project consisted of a 30 m stretch of a road pavement, 6 m wide. One half width of the road was constructed with HVFAC, and the other half was made with control concrete (without fly ash) for comparison purposes. Both the concretes were of M35 grade. In the first 13.5 m of the road, the right half width of the road was made with HVFAC and the left half width was made with control concrete. For the rest of the road, the location of the type of concrete was interchanged (Fig. 1). This is in order for both types of concrete to be subjected to the same conditions in terms of traffic load and sun exposure. Also, the first 6 m of the road was made with a thickness of 250 mm, and the rest of the road was made with a thickness of 200 mm (Fig. 1).
During concreting operations, strain gauges (vibrating wire type) were inserted at 63 critical locations for monitoring the temperature gradient in fresh and hardened concrete as well as stresses due to predefined loads. The actual stresses will be compared with the theoretical values obtained as per IRC 58.
The data will be used to modify the design codes for the concrete roads, which will be helpful in making cost-effective and efficient fly ash based concrete roads.
PARAMETERS STUDIED
The following are the parameters studied for the present project:
- Compressive strength as per IS 516 at 28, 56, 90 and 180 days
- Flexural strength as per IS 516 at 28, 56, 90 and 180 days
- Young's modulus of cylindrical samples at 28, 56, 90 and 180 days
- Coefficient of thermal expansion at 28, 56, 90 and 180 days
- Abrasion as per ASTM 779 by rotating disc-type equipment measured at 28, 56, 90 and 180 days
- Drying shrinkage as per ASTM 157 method at 28, 56, 90 and 180 days
- Chloride-ion penetrability was conducted as per ASTM 1202 on 28 and 56 days
- Temperature measurement in concrete up to 28 days
- Stress measurement with and without load on pavement under certain combinations, with temperature stresses at 28, 56, 90 and 180 days. The initial readings were taken with known load for calibration purpose, thereafter loading the pavement at various locations. A loaded truck was weighed for front axle and rear axle separately at a weigh bridge, and the weight of one wheel was determined with a load cell. The wheel load was transferred through a circular plate on the edge, corner, side and interior region of the pavement. The readings were taken in the daytime and at nighttime, with and without load for combining load stresses and temperature stresses.
- Stress distribution along panel and across the panel in HVFAC were studied under the following variables:
- Effect due to with/without use of polythene sheet between the concrete road and the lean concrete placed between the concrete road and the sub base road (Fig. 2)
- Effect on 200mm and 250mm thick pavement
- Effect of Span variation under 3m and 4.5m length
- Effect on edge stress due to side beams and without beam
- Performance of control concrete with HVFACSize of the panels and the road in mm.
Two cores of HVFAC and one from control concrete were also taken at 28 and 56 days and tested for strength.
The study continued up to 365 days, but the strain measurements can be done any time since the monitoring equipment is kept permanently on site. The data generated by the studies will be used for taking up issues related to concrete pavement.
A few photographs of the Greater Noida pavement are given below.
LOCATION OF STRAIN GAUGES
In each location given in the plan (Fig. 1), three strain gauges have been fixed. One at 25mm from the top, 25mm from the bottom and one in the centre. The horizontal distance of the gauge from the vertical face has been kept at 25 mm. For non-critical locations, two strain gauges have been fixed at the top and the bottom. Table 1 indicates the location and the number of strain gauges as well as the span and the thickness of the concrete panels.
Table 1: Number and location of strain gauges
Panel no.(Fig. 1)
|
Concrete type
|
Thickness, mm
|
Span, mm
|
Polythene sheets
|
Nos. of gauges
|
Side beam
|
1
|
HVFAC
|
250
|
3000
|
YES
|
NIL
|
YES
|
2
|
Control
|
250
|
3000
|
YES
|
NIL
|
YES
|
3
|
Control
|
200
|
3000
|
YES
|
1x2
|
YES
|
4
|
Control
|
200
|
4500
|
YES
|
1x3
|
YES
|
5
|
HVFAC
|
200
|
3000
|
NO
|
1x2
|
YES
|
6
|
HVFAC
|
200
|
3000
|
YES
|
NIL
|
YES
|
7
|
HVFAC
|
200
|
3000
|
YES
|
NIL
|
YES
|
8
|
HVFAC
|
200
|
3000
|
YES
|
NIL
|
YES
|
9
|
HVFAC
|
200
|
3000
|
YES
|
NIL
|
YES
|
1A
|
HVFAC
|
250
|
3000
|
YES
|
NIL
|
NO
|
2A
|
HVFAC
|
250
|
3000
|
YES
|
6X2
|
NO
|
3A
|
HVFAC
|
200
|
3000
|
YES
|
6X2
|
NO
|
4A
|
HVFAC
|
200
|
4500
|
YES
|
6X2+2X1
|
NO
|
5A
|
HVFAC
|
200
|
3000
|
NO
|
6X2
|
NO
|
6A
|
Control
|
200
|
3000
|
YES
|
NIL
|
NO
|
7A
|
Control
|
200
|
3000
|
YES
|
NIL
|
NO
|
8A
|
Control
|
200
|
3000
|
YES
|
NIL
|
NO
|
9A
|
Control
|
200
|
3000
|
YES
|
NIL
|
NO
|
On beam, cube samples for calibration
|
6
|
|
Total
|
63
|
CONCRETE MIXTURE PROPORTIONS
MATERIALS USED
Cementitious materials Cement OPC 53 grade and fly ash from Dadri thermal power station were used in this study. Their physical properties and chemical compositions are presented in Table 2.
Table 2: Physical properties and chemical analysis of the materials used
|
OPC cement Grade 53
|
Dadri fly ash
|
Physical Tests
|
|
|
Specific surface, Blaine, m2/kg
|
292
|
404
|
Particles retained on 45µ m sieve, %
|
-
|
15
|
Compressive strength of 51 mm cubes, Mpa
|
|
|
3-day
|
33.4
|
-
|
7-day
|
42.8
|
-
|
Lime reactivity, MPa
|
-
|
4.6
|
Soundness by autoclave, %
|
0.03
|
0.04
|
|
|
|
Chemical Analyses, %
|
|
|
SiO2
|
-
|
57.7
|
Al2O3
|
-
|
32.6
|
Fe2O3
|
-
|
3.3
|
SiO2 + Al2O3 + Fe2O3
|
-
|
93.6
|
CaO
|
-
|
2.5
|
Total Alkali
|
-
|
0.8
|
Magnesium oxide (MGO)
|
1.2
|
0.4
|
Sulphur trioxide (SO3)
|
2.6
|
Traces
|
Loss on ignition
|
1.0
|
0.6
|
Aggregates
Natural sand meeting the Indian standard requirements of zone II and crushed angular aggregates (20 mm and 10 mm) were used. The gradation of the sand and coarse aggregates is presented in Table 3.
Table 3: Grading of coarse and fine aggregates
Coarse aggregate
|
Fine aggregate
|
IS limits zone II
|
Sieve size, mm
|
CA 20 mm passing, %
|
IS limits passing, %
|
CA 10 mm passing, %
|
IS limits passing, %
|
Sieve size, mm
|
Passin g, %
|
Passing, %
|
40
20
12.5
10
4.75
|
100
99.2
-
0.1
0
|
100
85-100
-
0-20
0-5
|
-
100
99.1
64.5
0.6
|
-
100
100
85-100
0-20
|
4.75
2.36
1.18
0.60
0.30
0.15
|
97.5
91.3
68.6
47.7
21.2
5.7
|
90-100
75-100
55-90
35-59
8-30
0-10
|
Admixture
A naphtalene-based superplasticizer was used.
CONCRETE MIXTURE PROPORTIONS
Table 4 presents the mixture proportions of both HVFAC and control concrete used in the present project. Both concrete were designed to meet the requirements of M35 grade concrete.
Table 4: Concrete mixture proportions
|
M35 HVFAC
|
M35 Control
|
Cement, kg/m3
|
200
|
400
|
Fly ash, kg/m3
|
200
|
0
|
Water, kg/m3
|
128
|
135
|
Sand, kg/m3
|
625
|
689
|
Coarse agg., kg/m3
|
1205
|
1160
|
w/cm
|
0.32
|
0.34
|
Superplasticizer, %
|
0.8
|
2.5
|
CONSTRUCTION
PRODUCTION, PLACING AND FINISHING
The HVFAC was produced in a ready mixed concrete plant and was transported to the construction site using 6–m3 transit mixers. The concrete was placed in the forms and compacted using needle vibrators and levelled by a vibrating screed. The surface of the concrete was broom-finished to provide an anti-skid texture.
CURING
The surface of concrete was covered with plastic sheets for the first 24 hours to avoid evaporation and plastic shrinkage. Following that, wet gunny bags were used for curing for a period of 14 days.
RESULTS AND ANALYSIS
Strain Gauge Fixed on Side, Air Content Measurement
Fig. 3: Compaction with scareed vibrator , Fig. 4: Pouring from transit mixer
Fig. 5: Finishing of Concrete Pavement , Fig. 6: Edge Beam & Polythene Sheet
Fig. 8: Monitoring of reading from strain gauges , Fig. 7 : Joint cutting of HVFA concrete
FRESH PROPERTIES
Compressive strength
Table 5 shows the compressive strength test results up to 180 days for HVFAC and control concrete.
Table 5: Compressive strengths of HVFAC and control concrete
Age
|
Compressive strength, MPa
|
HVFAC
|
Control
|
3-day
|
12.1
|
29.7
|
7-day
|
18.7
|
46.2
|
28-day
|
36.7
|
51.3
|
56-day
|
46.4
|
60.6
|
90-day
|
53.6
|
60.5
|
180-day
|
58.2
|
60.4
|
As expected, HVFAC developed lower strength at early ages but higher strength rate development compared to those of control concrete. It was also expected that both kinds of concrete would achieve similar strength of around 40 MPa at 28 or 56 days, since both were designed to meet M35 Grade concrete. However, it appeared that the control concrete was over-designed, since it developed a 28- and
56-d strength of 51.3 and 60.6 MPa, respectively. For HVFAC, the strength was 36.7 and 46.4 MPa. at 28- and 56-d, respectively. At 180 days, both kinds of concrete developed similar strength of around 60 MPa. The results also show that the compressive strength of the control concrete reached a maximum at the age of 56 days, whereas that of HVFAC kept increasing even at 180 days.
Compressive strength determined on cores
Compressive strengths of HVFAC and the control concrete determined on cores are presented in Table 6.
Table 6: Compressive strength of HVFAC determined on cores
|
Compressive strength, MPa
|
28 days
|
56 days
|
HVFAC
|
38.4
|
42.8
|
Control concrete
|
47.2
|
-
|
- In the present project, the values determined on cores represented at least 92% of those determined on cubes.
Flexural strength
Table 7: Flexural strength of HVFAC and control concrete
Age
|
Flexural strength, MPa
|
HVFAC
|
Control
|
28-day
|
5.5
|
5.3
|
56-day
|
5.8
|
6.2
|
90-day
|
6.7
|
6.3
|
180-day
|
7.5
|
6.3
|
The results presented in Table 7 show that HVFAC developed a flexural strength of 5.5 MPa at 28 days, which is similar to that of the control concrete. In line with the compressive strength development of both concretes, the flexural strength of HVFAC kept increasing with time to reach 7.5 MPa at 180 days, whereas that of the control concrete reached a maximum of 6.2 to 6.3 MPa at 56 days. These results show the benefits of using HVFAC in road pavements.
Abrasion resistance
The depth of abrasion at different ages of HVFAC and control concrete is presented in Table 8.
Table 8: Abrasion resistance of HVFAC and control concrete
Age
|
Depth of abrasion, mm
|
HVFAC
|
Control
|
28-day
|
0.67
|
0.47
|
56-day
|
0.66
|
0.51
|
90-day
|
0.59
|
0.63
|
180-day
|
0.60
|
0.35
|
Published data show that abrasion resistance is closely related to the compressive strength of concrete [2]. The type of aggregate and surface finish or treatment used also has a strong influence on abrasion resistance. Fly ash generally does not affect this property beyond its influence on strength and finishing. Since Table 5 has shown higher compressive strengths for the control concrete at early ages and similar strength at 180 days compared to those of HVFAC, it was expected to have the same trend for abrasion resistance. However, the above results show that the control concrete had higher abrasion resistance than HVFAC at all ages, but the depth of abrasion was generally low for both concretes.
Modulus of elasticity
Table 9: Modulus of Elasticity of HVFAC and control concrete
Age
|
Modulus of elasticity, GPa
|
HVFAC
|
Control
|
28-day
|
33.5
|
39.5
|
56-day
|
33.7
|
40.7
|
90-day
|
34.6
|
39.0
|
180-day
|
35.4
|
39.9
|
HVFAC developed a modulus of elasticity of 33.5 and 35.4 GPa at 28 and 180 days respectively; for the control concrete, these values were 39.5 and 39.9 GPa (Table 9). The results show that the increase with time in the modulus of elasticity was slightly higher for HVFAC than for the control concrete. However, the modulus of elasticity of HVFAC appeared to be lower than that of control concrete at all ages.
Drying shrinkage
Table 10 presents the drying shrinkage of HVFAC and control concrete at different ages.
Table 10: Drying shrinkage of HVFAC and control concrete
Age
|
Drying shrinkage, %
|
HVFAC
|
Control
|
28-day
|
0.0240
|
0.0356
|
56-day
|
0.0240
|
0.0260
|
90-day
|
0.0200
|
0.0220
|
180-day
|
0.0189
|
0.0200
|
The results show that the drying shrinkage of HVFAC was lower than that of the control concrete at all ages. This might be due to the lower water content of HVFAC compared to that of the control concrete.
These results suggest that for HVFAC the size of the panels may be bigger and the cost of the thermal joints may be reduced. However, this needs to be proved.
Coefficient of thermal expansion
The coefficient of thermal expansion of HVFAC and the control concrete at different ages is presented in Table 11.
Table 11: Coefficient of thermal expansion of HVFAC and control concrete
Age
|
Coefficient of thermal expansion, 10-6/°C
|
HVFAC
|
Control
|
28-day
|
13.2
|
11.5
|
56-day
|
11.6
|
9.9
|
90-day
|
12.8
|
11.3
|
180-day
|
12.8
|
11.9
|
However, the difference between the coefficients of thermal expansion of both concretes was not critical. In fact, it is lower than the difference between that of a conventional concrete made with siliceous aggregates (11.3 to 12.6 10-6/°C) and that of a conventional concrete made with calcareous aggregates (5.4 to 9.0 10-6/°C) [3].
Chloride-ion penetrability
Table 12: Chloride-ion penetrability of HVFAC
Age
|
Chloride-ion penetrability, Coulombs
|
HVFAC
|
Control
|
28-day
|
870
|
1060
|
91-day
|
170
|
-
|
HVFAC developed very low chloride-ion penetrability at both 28 and 91 days (Table 12). This is mainly due to high volume fly ash content and very low w/cm. This shows that this type of concrete will potentially provide good protection to the reinforcing steel by preventing chloride-induced corrosion. The control concrete developed a Coulombs value that is very close to very low chloride-ion penetrability at 28 days. This was mainly due to the low w/c of this concrete (Table 4).
Stress measurements
Preliminary stress measurements due to load and temperature have been taken, and the data is under processing. The preliminary test results of strains, temperature gradient and deflection are showing better performance of high volume fly ash concrete as compared to control concrete. A detailed analysis of the data will throw more light on the beneficial effects of the new technology pavement made with HVFAC.
SUGGESTED COST REDUCTION MEASURES
Fig. 9: Abrasion test as per ASTM 799C , Fig. 10: Load test
The benefits of the concrete road in terms of better performance and lower life-cycle cost have been very well accepted by the engineering fraternity. However, the initial cost of the concrete road becomes a constraint due to limited funds as compared to the bitumen road. The cost of the concrete road can be reduced by adopting the technology of HVFA concrete, by using fly ash and making the concrete environmentally greener. The main parameter contributing the higher thickness of the concrete road is the combined effect of load and temperature stresses. At present, a slab thickness of 400-450 mm is being used, which can be effectively reduced to 300–350 mm for highways and 250–300 mm for other roads. By adopting the following measures, the cost of concrete roads can be reduced. An experimental study has been undertaken by ACC at Greater Noida to measure the performance of the road under following conditions by measuring the actual stresses by inserting strain gauges (vibrating wire type) at various depths and locations of concrete pavement.
1. Widened outer layer: The widened outer lane as a shoulder or a concrete shoulder, which is jointed properly with tie bars, will effectively reduce the stresses, and the thickness of main pavement can be reduced for economy.
2. Beam on the edge: The edge stress is the main governing criteria in the pavement design. If a
reinforced beam of matching thickness on the edge is cast as a hidden beam along with the pavement, then the major part of the edge stresses will be taken care of by the hidden beam, and the overall pavement thickness can be reduced to a great extent. Since the reinforcement cage of the beam is laid in advance on the side before pouring concrete, paver operations are not affected.
3. Reduced joint spacing: The longer spacing of transverse joints means higher stiffness ratio and greater thickness of the pavement. If the spacing is maintained between 3.0m to 4.5m, the pavement thickness can be reduced. This will also reduce the transverse cracking, which is the one of the main causes of pavement failure.
4. Combining PQC and DLC layer: This can be achieved by eliminating the plastic sheet layer to establish a strong bond between DLC and PQC. This requires matching joints in the DLC and PQC and needs care during construction for providing base joints in DLC, curing of DLC, in matching joints of DLC and PQC, timeliness of joint cutting of PQC, etc.
5. Designing PQC for 90 days strength in place of 28 days strength: Concrete roads are being constructed in long stretches or in panels in small segments. It is very rare that the roads are opened to traffic immediately after 28 days. Hence, the concrete can be designed for 56 days or 90 days flexural strength instead of 28 days. The % gain in flexural strength in PPC or HVFA concrete is much higher than the OPC concrete. This will substantially bring down the thickness of the road and hence the cost, which is the main concern in the construction of the concrete road.
CONCLUSIONS
It have been observed that the fly ash replacement levels up to 50% can produce a good concrete of M40 grades with good one-day compressive strength for de-shuttering, thus out compromising the speed of construction. It has also been observed that the flexural strength increases to 40 – 55 % between 28 days and 90 days for HVFA concrete and this property can be used for designing the concrete pavement based on 90 days flexural strength.
Studies being conducted at Greater Noida will help in understanding the performance of the concrete pavement under various parameters like comparison of theoretical stresses as per design and actual stresses on site due to load, temperature and a combination of both, effect of side beams on edge stress, effect of eliminating the plastic sheet between DLC and PQC layer and effect of shorter panel length. Research agencies and interested individuals are requested to further extend the studies on the theoretical and actual stresses due to loadings on different conditions like panel size, effect of polythene sheet between DLC and PQC, effect on HVFAC and the control concrete, effect of edge beam, etc. This will help in reducing the thickness of pavement since many people feel that we are over-designing the concrete thickness by using the empirical formulae of the Westergaard equation given in IRC-58 2002. The data on the actual temperature gradient and the resultant stresses is being generated for better understanding and designing the pavement in a scientific manner instead of following the empirical formulae.
An HVFA concrete pavement performs well under heavy traffic, withstands temperature effects and moisture movement better than normal concrete and has shown better abrasion resistance. The initial cost of an HVFAC road is 15- 25% lower than the normal concrete road and only 5 –10% more than the cost of a bitumen pavement, depending upon the cost of local aggregates, which form the bulk of the cost. In case of non-availability of good quality fly ash and an RMC plant at construction site, it is advisable to use fly ash–based portland pozzolana cement in place of OPC to get the advantages of better abrasion resistance and higher flexural strength over a period of time.
ACKNOWLEDGEMENTS
The guidance received during the execution of the above project from Mr P. Srinivasan, ACC, Dr L. R. Kadiyali and Dr Venketesh of Kadiyali and Associates, Mr Benoit Fournier of CANMET and Mr R. C. Wasan, NCBM, is duly acknowledged.
REFERENCES
- Malhotra V.M. and Mehta P. K. - High-Performance, High Volume Fly Ash Concrete: Materials, Mixture Proportioning, Properties, Construction Practice and Case Histories
- Dr. L. R. Kadiyali - Detailed Project Report on HVFC Road in Gauriganj, UP
- IRC 58-2002 and IRC 15-2002
- Banchhor Anil - High Volume Fly Ash RCC Building - First of its kind in India - National Conference on High Volume Fly Ash Concrete by CANMET, Canada Dec 2005
- Banchhor Anil - Performance Evaluation of Concrete made with Factory made PPC and Site mixed Fly Ash Concrete - 9th NCB International seminar, Delhi, Nov 2005
- Banchhor Anil, Srinivasan, P and Tiwari A. K.- Suitability of HVFA Concrete for Pavements
- Banchhor Anil - Green Concrete Pavement, TIFAC seminar at Allahabad 2005
- Binod Kumar, Sood V. K. and Bose Sunil - Effect of Non-linear temperature gradient on maximum temperature stresses in rigid pavements Indian Concrete Journal, Sept. 2004
- Naik, T. R. & Ramme, B. W., Long Term Performance of High Volume Fly Ash Concrete Pavements, Report No. CBU - 2002 -29, October 2002
- 10. Malhotra, V. M., High-Performance HVFA Concrete: A Solution to the Infrastructural Needs of India", Indian Concrete Journal, February 2002.