An Example of Magnificent Integral Gothic Architecture
Burgos Cathedral, Spain
Burgos Cathedral is a Gothic-style Roman Catholic cathedral in Burgos, Spain. The construction of a cathedral at Burgos was ordered by King Ferdinand III of Castile and Mauricio, the English-born Bishop of Burgos. Construction started on the site of the former Romanesque cathedral on July 20, 1221. Work began at the chevet (east end) which was completed in nine years.
The architect's principal responsible for its construction was a Frenchman named Enrique (who also worked on Leon Cathedral) in the 13th century and a German named John of Cologne (Juan de Colonia) in the 15th century. The latter was discovered and hired by the bishop of Burgos while attending the Council of Constance in 1417.
The high altar was consecrated in 1260 and then there was a lengthy hiatus of almost 200 years before construction started up again. The cathedral was finally completed in 1567 with the addition of the lantern spire over the main crossing (which rises above a delicate openwork star vault). In 1919 the cathedral became the burial place of Rodrigo Diaz de Vivar ("El Cid"), and his wife Dona Jimena. On October 31, 1984, Burgos Cathedral was designated a World Heritage Site by UNESCO.
The Collapsed Structure
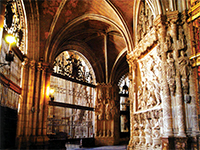 |
The Cathedral of Burgos is situated at the centre of historic centre of Burgos. The entire complex is supported on the slope of the citadel hill and a series of underground chapels. The main church is a Latin cross in plan, arranged in a nave and two aisles. Longitudinally, the church is enclosed and fixed by a facade characterised by a rich decoration that incorporates deep buttresses and spires that crown the two towers.
|
Highly decorated facade structures that integrate stiffening buttresses and turrets at their corners perform a similar retaining function for the transepts as well. A freestanding octagonal tower marks the crossing and is supported on four heavy piers. The zone of the apse is where most of the construction phases can be identified. A first ring of radial chapels was added after the Renaissance and traces of other lesser chapels can still be seen at the external perimeter of the apse. The entire Cathedral is flanked by chapels, most of which have been accommodated in various configurations and styles between the outer pier buttresses.
The crossing tower is a focal point of the scheme not only because of its position, volume and decoration but also due to the major reconstruction of the entire crossing area its collapse caused in 1539. The heavy pilasters that were then incorporated provide further stiffness to the ends of the choir and the nave and to some extent compartmentalise structurally the building.
The Tasde-Charge
The elevation of the nave follows the typical division into nave arcade, triforium and clerestory and the differences with that of the choir are mainly stylistic. The compound piers are formed by a cluster of 8 shafts that join the ribs of the vaults. The nave arcade is a pointed arch with a not very deep section, which means the tasde- charge ending of the wall above has a large offset.
At the interior, the springing of the nave vaults meet over a wider area and thrust is spread more efficiently around the head of the rampant arch with the help of rubble filling the conoid pockets behind the spandrel walls.
The structural behaviour of the Cathedral can be simplified to that of a typical bay of the nave as there are sufficient transverse structures that function as diaphragms, isolating parts of the building into areas with an almost uniform stress regime. The third bay from the facade appeared to be quite representative in terms of structural layout and support decoration.
Construction Duties
The most important phase in the development of the Cathedral is the foundation and construction of the original design that succeeded a previous Romanesque cathedral. On 20 July 1221 the foundation stone was laid and with interruptions. The main fabric was completed in 1277.
The next major phase (1446-1568) was characterised by a well-known family of German master masons (Juan, Simon and Francisco de Colonia). They were responsible for most of the style of the Cathedral as they designed the spires in fine tracery at the west facade, built the crossing tower and redesigned some of the major chapels. The original crossing tower collapsed after a tempest in 1539 and was reconstructed by 1568.
The establishment of the major chapels was concluded in 1736 with S. Thecla (behind the north side of the facade). Stylistic changes and conservation works started with the reformation of the facade in 1753 and major works took place in 1885-1900 by R. Velazquez-Bosco and V.Lamperez.
Behaviour of the Nave
The model was generated with a CAD pre-processor and then solved with the FE program Abaqus. Due to symmetry only a quarter of the bay was examined and 3D "brick" type elements were used. The material was initially considered as linear elastic and orthotropic properties were assigned to the webs of the vaults.
A linear elastic analysis was performed under self weight of the materials. The resulting deformation of the FE model and the stresses are generally low and it indicates the efficiency of the design of the building, in response to the high compressive strength of the constitutive materials and elements.
Vault Structure
The nave vault can be simulated as a parabolic barrel vault running along the main axis of the nave, stiffened at the edges with the deeper transverse vault. As in these proportions the parabolic profile is close to the catenary, the hoop stresses S22 are low, despite the presence of low tension. The associated high thrusts are balanced by the flying buttresses and the vault is further stiffened by the transverse vault due to its circular profile and stilted arrangement.
However, the central portion of the vault still tends to spread outwards. The stiffer transverse web transmits most of this horizontal deformation onto the wall and tension develops there mainly at the area between the vault and the crown of the clerestory window. As a consequence, compression results along the straight vertex of the transverse vault which diminishes closer to the wall.
The relative stiffness of the transverse web is further demonstrated by the mainly compressive low hoop stresses. The increasing values at the haunches indicate a part of the weight of the vault is indeed supported by the rib and subsequently the springing. The associated thrust twists the longitudinal web, causing longitudinal tension at most part of the area.
The rib is a quite crucial element as it guarantees the good execution of the critical area of the intersection as well as the continuity between the webs. As a consequence, the sharp change in the curvature stiffens each of the adjoining webs but also functions as a barrier against the spread of high stresses between the webs. The supports in each of these vaults vary in their stiffness between the fixed transverse edge on the wall and the flexible symmetric boundary of the longitudinal webs.
The Triforium Wall
The transition from the section of the wall at the triforium to the narrower section above the arcade pier is the critical area of the tas-de-charge (Fitchen 1961). Apart from the loads of the nave being transmitted through, further difficulties arise from the need to fix the roof that protects the aisle vault.
The behaviour of the lateral wall can also be illustrated by the deformation of the pier extension, in particular the shaft from the compound pier that runs continuously until the crown.
However, further above, the inward displacement at the middle of the nave arch highlights the effect of the thrust of the flying buttress on the nave, although the values are relatively small. In order to show the proportions of the problem, a comparison is made with the heavily deformed bays of Santa Maria la Vieja, the Old Cathedral of Vitoria.
The deformations were recorded through a monitoring programme and are attributed to the lack of flying buttresses from the original project and their belated addition once the nave of that Cathedral had started spreading.
Lower Structure
The main actions on the aisle vault are the self weight of the masonry and the loads from the upper structure applied asymmetrically over the arcade. The vault is contained between the pier arcade and the pier buttresses and therefore the lateral thrusts are not contained in the same symmetrical manner as in the nave vaults. Both vertices are curved and the domical pattern was probably chosen intuitively to reduce the bend. The nave arch and its immediate area receive directly the load from the upper structure through the tas-de-charge. The load twists the vault and pulls it from the adjacent vault along the transverse edge making the increase the transverse rib causes in the stiffness of the edge quite important.
Cracks and Cutting
The torsion affects the entire vault and a similar pattern develops in the other direction as well. If the two patterns are examined together, an in-plane, shear deformation appears to develop due to the torsion of the vault. The rotation can be associated with the deformation of the upper structure due to the apparently excessive thrust from the flying buttresses observed earlier. The lateral wall seems to have sufficient stiffness to transmit most of this deformation directly on the aisle.
The domical layout of the aisle vault has not only reduced the deformations but also enhanced the two way behaviour of the vault, making the loads to flow into the entire area of the supports. The diagonal ribs are now more organically bonded into the webs and, since the groins are smoother, their function is now mainly to increase the depth of the section and therefore reduce the deformations.
The tensile forces however are close to the modulus of rupture Fu and cracks are anticipated now on the apex of the edge of the vault on the wall and at the front haunches. Another benefit of this pattern is therefore that the cracks along the longitudinal vertex that develop in vaults with straight vertices are not expected to affect this vault. A closer inspection of the aisle vaults shows that there have been repairs at the past mainly at these parts at the haunches.
The combination of the in-plane rotation of the vault with the eccentrically transmitted vertical loads of the upper structure is somehow beneficial for the arcade pier that ultimately supports these loads. Despite the relatively higher compressive stresses, the variation of the vertical loads in the section is not strong and they appear to be transmitted axially.
The weight of the pier has managed to reduce significantly the overturning effect of the thrusts from the flying buttresses and the aisle. The bending stresses due to the offset with which these forces are transmitted to the ground are contained within the limits of strength.
Load Bearing Elements
The lateral displacement of the bay has increased as the higher inward deformation of the nave vault. The values however do not compare with the magnitude of the deformations observed in Vitoria Cathedral, which must have been affected by time-dependent decay of the joints and the incorrect location of buttressing systems once the deformations became appreciable. The range of horizontal displacements that is expected to develop at the springing of a vault can be accessed from available survey data on the deformation of the lateral walls of some cathedrals.
Another interesting area to be investigated is the behaviour of the cross vaults. The aisle cross vault was modelled separately in more detail and both the webs and the ribs and arches were generated by shell elements, following a method outlined in (Theodossopoulos 2003). At this model, the nave arch is sufficiently stiff to provide an almost symmetrical support to the vault.
Burgos Cathedral demonstrated the success of the original design of the building as the stresses were overall below the strength of the constituent materials and the deflections were quite low. The quality of the original design is also evident in the load-bearing elements of the Cathedral as they proved to make an efficient use of the strength of the stone masonry in compression while reducing tensile stresses to a minimum possible.
The model highlighted the interaction between the upper and lower structure as well as the factors that influence the flow of stresses towards the supports. The rib plays a key role in the execution of the difficult intersection between the webs and the efficient distribution of the loads within the entire
vault according to a two-way pattern.
References:
http://whc.unesco.org/pg.cfm?cid=31&id_site=316
http://designspeculum.com/Historyweb/thebur.pdf
http://famouswonders.com/burgos-cathedral/
http://www.caminomyway.com/my-visit-to-the-burgos-cathedral