Earthquake-Resistant Construction of Adobe buildings
A tutorial By Marcial Blondet, Gladys Villa Garcia M, Svetlana Brzev, Alvaro Rubinos
Adobe mud blocks are one of the oldest and most widely used building ma- terials. Use of these sundried blocks dates back to 8000 B.C. (Houben and Guillard 1994). The use of adobe is very common in some of the world's most hazard-prone regions, such as Latin America, Africa, the Indian subcontinent and other parts of Asia, the Middle East and Southern Europe, as shown in Fig- ures 1.1 and 1.2.
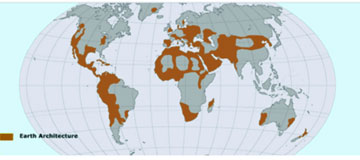
|
Figure 1.1 World distribution of earth architecture (source: De Sensi 2003) |
Around 30% to 50% of the world's population (approximately 3 billion people) lives or works in earthen buildings. Approximately 50% of population in developing countries, including a majority of the rural population and at least 20% of the urban population, live in earthen dwellings (Houben and Guillaud 1994). For example, in Peru, according to the 2007 Census, almost 40% of houses are made of earth (that's 2 million houses inhabited by around 9 million people). In India, according to the 2001 Census, 30% of all buildings are made out of earth (this in- cludes 73 million houses inhabited by almost 305 million people).
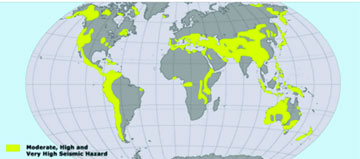
|
Figure 1.1 World distribution of moderate and high seismic risk (source: De Sensi 2003)
|
Adobe construction is mainly used in rural areas. Houses are typically one-story high, with wallheights of around 3.0 m and thicknesses rangingfrom 250 mm to 850 mm. In mountainous re- gions with steep hillsides such as the Andes, houses can be up to three stories high. In parts of the Middle East, earthen houses are often built one on top of the other, so that the roof of one house is used as the bottom floor of the house above. Adobe houses are found in the urban areas of most developing countries. In some countries, like Argentina and Chile, and in some cities, like San Salvador, adobe construction is banned by building codes because of its poor seismic performance (Blondet and Villa Garcia 2004). Typical adobe houses featured in the World Housing Encyclopedia (www.world-housing.net) are presented in Figure 1.3.
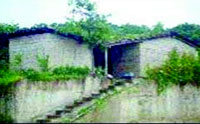
|
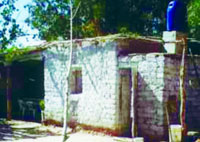
|
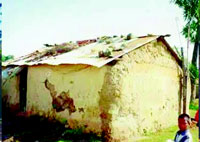
|
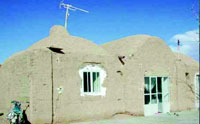
|
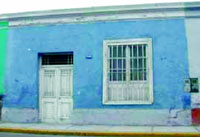
|
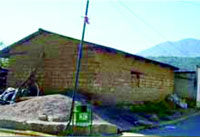
|
Figure 1.3 Typical adobe houses around the world: a) EL Salvador (source: Lopez et al.2002, b) Argentina (Source: Rodriguez et al 2002), c) India (Source: Kumar 2002), d) Iran (Source: Mehrain and Naeim 2004), e) Peru (Source: Loaiza et al. 2002), and f) Guatemala (source: Lang et al. 2007) |
Adobe is a low-cost, readily available construction ma- terial, usually manufactured by local communities, as shown in Figure 1.4. Adobe structures are generally made by their owners because the construction practice is simple and does not require additional energy resources. Skilled technicians (engineers and architects) are generally not involved in this type of construction, hence the terms "non-engineered construction" and "informal construction".
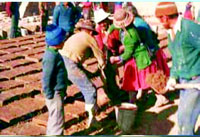
|
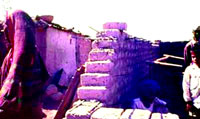
|
Figure 1.4 Adobe Construction performed by local communities: a) Block making in peru (photo: M. Blondet), and b) Building construction in India (photo: S. Brzev)
|
Earthquake Performance
In addition to its low cost and simple construction technology, adobe construction has other advantages, such as excellent thermal and acoustic properties. However, adobe structures are vulnerable to the effects of natural phenomena such as earthquakes, rain, and floods. Traditional adobe construction responds very poorly to earthquake ground shaking. The seismic deficiencies of adobe buildings are caused by its heavy weight, low strength, and brittleness. During earthquakes, these structures develop high levels of seismic forces they are unable to resist, and often fail abruptly.
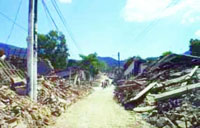
|
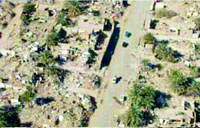
|
Figure 2.1 Collapsed adobe buildings: a) 2001 El Salvador earthquake (photo: D. Dowling). and b) 2003 Bam, Iran, earthquake (source: Mehrain and Naeim 2004) |
Considerable damage and loss of life has occurred in areas where adobe has been used. In the 2001 earthquakes in El Salvador, 1,100 people died, more than 150,000 adobe buildings were severely damaged or collapsed (Figure 2.1a), and over 1.6 million people were affected (Dowling 2004a). That same year, the earthquake in the south of Peru caused the deaths of 81 people. These deaths can largely be attributed to the 25,000 adobe houses that collapsed and the 36,000 that were damaged.
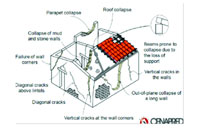 |
Over 220,000 people were left without shelter (USAID Peru 2001). In the 2003 Bam earthquake in Iran, more than 43,000 people died and over 60,000 were left without shelter, primarily due to the collapse of adobe buildings (EERI 2004), as shown in Figure 2.1b. The 2007 Pisco, Peru earthquake destroyed more than 75,000 dwellings. More than 600 people died and another 300,000 were affected by the earthquake (INEI 2007). Adobe buildings were also damaged in the rural areas affected by the 2008 Wenchuan, China earthquake (EERI 2008) and the 2010 Maule, Chile, earth- quake (Astroza et al. 2010). |
Figure 2.2 Seismic deficiencies of adobe buildings (CENAPRED 2000)
|
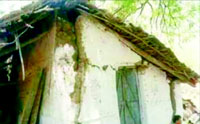
|
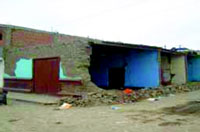
|
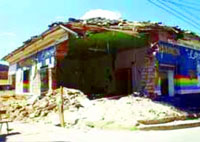
|
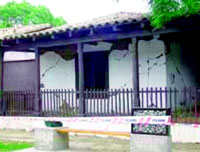
|
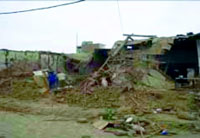
|
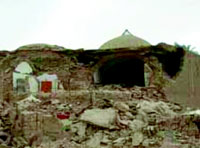
|
Figure 2.3 Typical patterns of earthquake damage in adobe walls: a) Vertical cracking and separation of adobe walls after the 1997 Jabalpur, India earthquake (source: Kumar 2002), b) Out-of-plane wall collapse after the 2007 Pisco, Peru earthquake (photo: M. Blondet), c) Total collapse of adobe walls after the 2001 El Salvador earthquake (source: Lopez et al. 2002), d) Diagonal cracking of adobe walls after the 2010 El Maule, Chile earthquake (source: Astroza et al. 2010), e) Roof collapse on an adobe building after the 2007 Pisco, Peru earthquake (photo: M. Blondet), and f) Parapet collapse on an adobe building after the 2003 Bam, Iran earthquake (source: Mehrain and Naeim 2004) |
Typical earthquake damage patterns for adobe buildings include vertical cracking and separation of walls at the corners, diagonal cracking in the walls, and out-of- plane wall collapse. Separation of roofs from walls in buildings without adequate wall-to-roof connections often leads to complete building collapse. Damage patterns characteristic of adobe construction are summarized in Figure 2.2 and typical damage patterns observed in past earthquakes are shown in Figure 2.3.
Improved Earthquake Performance of New Adobe Construction
Due to its low cost, adobe construction will continue to be used by impoverished people in many regions of the world, including those regions with high seismic risk. The implementation of cost-effective building technologies to improve the seismic performance of adobe buildings is critical to achieving seismic safety for a substantial portion of the global population. Based on state-of-the-art research and observations from past earthquakes, the key factors for improving the seismic performance of adobe construction are:
- Adequate soil properties and construction quality
- Wall construction
- Robust layout
- Use of improved building technologies with seismic reinforcement
Adequate Soil Properties and Con-struction Quality*
The soil properties that have the greatest influence on the strength of adobe masonry are those related to the dry strength of the material and the drying shrinkage process, as further:
- Clay is the most important component of the soil used for adobe construction. It provides dry strength, however it also causes drying shrinkage of the soil.
- Controlled microcracking of the soil mortar due to drying shrinkage is needed for strong adobe masonry construction. Straw and, to a lesser extent, coarse sand are additives that control the micro- cracking of the mortar due to drying shrinkage, and therefore improve the strength of adobe masonry.
- The quality of construction plays an important role in creating strong adobe masonry, resulting in broad strength variations up to 100%.
A review of tests for selecting adequate soil for earth construction was performed by Neves et al. (2009). The most relevant field tests and recommendations are summarized below.
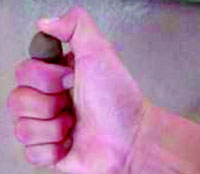
|
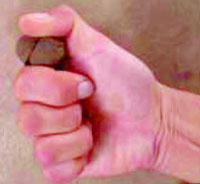
|
Figure 3.1 Dry strength test: a) Adequate soil, and b) Inadequate soil
|
The "dry strength test" consists of making at least five mud balls with a diameter of about 20 mm from the selected soil. After the balls have dried for at least 24 hours, try to crush each ball between the thumb and the index finger, as shown in Figure 3.1. If none of the balls can be broken, the soil con- tains enough clay to be used for adobe construction (provided that microcracking of the mortar due to drying shrinkage is controlled). If some of the balls can be crushed (Figure 3.1b), the clay content is insufficient and the soil is inadequate.
The "roll test" consists of making a mud roll with a diameter of about 20 mm. Roll the mud using both hands and hold it vertically by the end so that it is hanging freely, as shown in Figure 3.2. If the continuous roll length is between 50 mm and 150 mm, the soil is adequate for use in adobe construction. If the roll breaks at less than 50 mm long, the soil is inadequate. If the roll breaks at more than 150 mm long, coarse sand must be added to the soil.
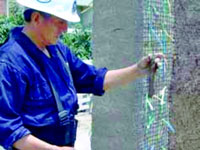 |
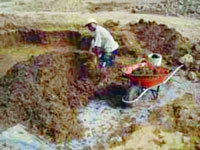 |
Figure 3.2 Roll Test |
Figure 3.3 Adding straw to the soil: a) Straw to be added, and b) Mixing the straw and soil
|
Adding straw to the soil controls the microcracking effect caused by drying shrinkage. The maximum amount of straw added to the soil should still allow adequate workability. Figure 3.3 shows how straw can be added during the preparation of the soil.
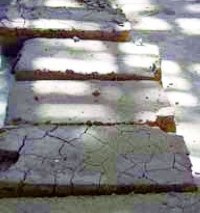 |
Coarse sand can also be used to control microcracking due to drying shrinkage. The best proportion of soil and coarse sand can be determined by performing the "microcracking control test". A minimum of four "sandwiches" made of two adobe bricks joined with mortar need to be made using mortars with different proportions of soil and coarse sand (approximate particle size 0.5 mm to 5 mm). It is recommended that the soil-to-coarse sand proportions vary between 1:0 (no sand) to 1:3 in volume. The sandwich with the least amount of sand that shows no visible cracking when it is carefully opened after 48 hours indicates an adequate soil-to-coarse sand proportion for mortar in adobe construction. Figure 3.3 shows sandwiches with visible cracking. |
Fig 3.3 Opened "sandwiches" with visible cracking
|
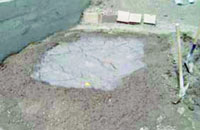 |
"Sleeping" the mud means leaving the soil with water for one day before preparing the mud and making the adobe bricks or mortar Figure 3.4). This procedure, traditionally followed in Peru, improves the integration and distribution of water with the clay particles, thus activating their cohesive properties.
Wetting the adobe bricks prior to the construction is a good practice. All adobe surfaces should be wet. This can be achieved by soaking adobe bricks in water for about 5 seconds, as shown in Figure 3.5.
Other construction recommendations include:
- Foreign matter should be removed from the soil.
- Mud should be mixed thoroughly and uniformly.
- Adobe bricks should be dried in the shade.
- Bricks should be cleaned before wetting and laying.
|
Fig 3.4 "Sleeping" the mud |
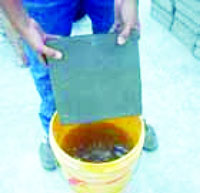 |
Fig 3.5 Wetting an adobe brick |
|
Wall Construction
This section provides some key recommendations related to the construction of adobe walls.
A foundation made of concrete (Figure 3.6a) or brick masonry should be built to provide damp- proofing for adobe walls. A liquid asphalt layer applied at the surface of the foundation before the wall construction increases damp-proofing, as show in Figure 3.6b.
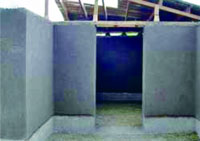
|
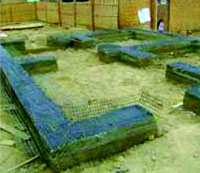
|
Figure 3.6 Protection of adobe walls against damp: a) Adobe walls with a concrete foundation, and b) Liquid asphalt on a foundation |
Horizontal and vertical mortar joints should be uniform and completely filled, in order to make strong adobe masonry.
The adobe walls should be covered with mud plaster. Plaster increases the stiffness and the strength of adobe walls and provides environmental protection.
Robust Layout
One of the essential principles of earthquake-resis- tant adobe construction is the use of a compact box- type layout (Figure3.7).
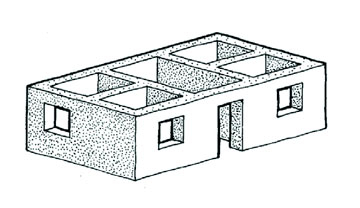
|
Figure 3.7 The safest building form is a squat, single-story house, with small windows and a regular, compact plan with frequent cross-walls (source: Coburn et al. 1995) |
- Build only one story high.
- Use an insulated lightweight roof instead of a heavy compacted earth roof.
- Select a wall layout that provides mutual support by cross walls and intersecting walls at regular inter- vals in both directions (alternatively, buttresses can be used).
- Keep openings in the walls small, centered, and well-spaced.
- Build on a firm foundation.
- Walls are the main load-bearing elements in adobe buildings. A number of empirical recommendations regarding earthquake-resistant wall construction are as follows (Figure 3.8):
- The wall thickness should be at least 400 mm.
- The wall height should not exceed 6 times the wall thickness at its base, and in any case should not be greater than 3.5 m.
- The unsupported length of a wall between cross walls should not exceed 10 times the wall thickness, with a maximum of 7.0 m.The length of wall openings should not exceed one-third of the total wall length.
- The length of openings should not exceed 1.2 m.
- The length of piers between openings and the distance from cross-walls to openings should be at least 1.2 m.
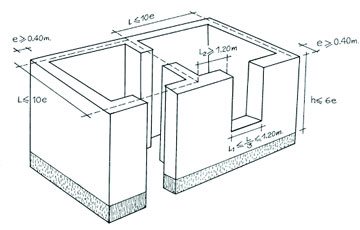
|
Figure 3.8 Empirical recommendations regarding earthquake-resistant wall construction (source: Blondet 2010) |
Seismic Reinforcing Systems for New and Existing Adobe Construction
This section is mostly based on research performed at the Catholic University of Peru (PUCP) in the past 40 years. These research studies are described in several publications (Bariola et al. 1986, Blondet et al. 2002, PUCP/CIID 1995, Vargas et al. 2005). Reinforcing systems described below can be used for both new and existing adobe construction. Guidelines on adobe construction have been developed in several countries (e.g., IAS 2004). For a comprehen- sive overview of research studies and guidelines for adobe construction in Latin America the reader is referred to Papanikolaou and Taucer (2004).
Ring Beams*
Ring beams (also known as a crown beams, collar beams, bond beams, tie-beams, or seismic bands) are one of the most essential earthquake-resistant provisions for load-bearing masonry construction. A ring beam ties the walls together and ensures that the building behaves like a box when subjected to earthquake ground shaking (a box effect is critical for satisfactory earthquake performance). The ring beam must act like a belt and must be strong, continuous, and securely tied to the walls. It must be placed atop all walls, its width should match the wall width, and it must provide support for the roof. The ring beam can be made of wood, eucalyptus logs, or bamboo. Figure 4.1 shows ring beams made of different materials.
A wooden ring beam (Figure 4.1a) is built using long timber members and transverse pieces nailed at 400 mm spacing. The wooden beam is made of 75 mm by 75 mm joints. A ring beam made of euca- lyptus logs is shown in Figure 4.1b and a bamboo ring beam is shown in Figure 4.1c. The diameter of the elements for eucalyptus or bamboo ring beams should be about 100 mm to 120 mm. All the joints must be nailed together and firmly tied with galvanized steel wire.
|
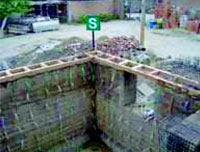 |
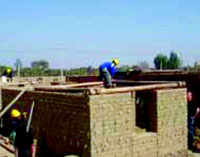 |
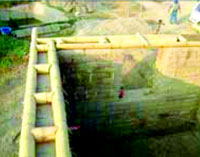 |
Figure 4.1 Ring beams made of different materials: a) Wood, b) Eucalyptus logs, and c) Bamboo
|
Wall Reinforcement Schemes
During an earthquake, adobe walls are subjected to tensile stresses that they are unable to resist, thus cracks appear and the walls break into large pieces. This damage can lead to either the partial or total collapse of the structure. To prevent this, vertical and horizontal seismic reinforcement must be placed at critical locations. The reinforcement must be continuous and can be either inside the wall or attached to the wall surface.
Vertical reinforcement ties the wall to the foundation and the ring beam, and it also restrains the out-of-plane bending and in-plane shear effects. Horizontal reinforcement transmits the bending and inertia forces in transverse walls (out-of-plane) to the supporting shear walls (in-plane). It also restrains the shear stresses between adjoining walls and minimizes vertical crack propagation. Horizontal and vertical reinforcements should be properly tied to one another and also connected to the adjoining structural elements (the foundation, ring beam, and roof ). This reinforcing scheme results in a stable 3-D structure.
Reinforcement can be made using various mate- rials compatible with adobe masonry, including bamboo, reeds, cane, vines, rope, timber, PVC tubes, steel bars, barbed wire, steel mesh, plastic mesh, and polymer mesh. More detailed descriptions of these reinforcement schemes and their effectiveness can be found below. The results of recent PUCP research studies suggest that the most effective reinforcement is polymer mesh surrounding all adobe walls.
Internal cane reinforcement
Several research studies on adobe buildings with cane reinforcement have been performed at PUCP (Blondet et al. 2002). In an experimental research study performed in 1972, adobe building models were built on a concrete platform. The testing consisted of slowly tilting the platform and measuring the tilt angle at collapse (Figure 4.2). The lateral component of the weight of the model was then used to quantify the maximum seismic force. It was concluded that internal vertical reinforcement made of cane, combined with horizontal crushed cane placed in the wall at every fourth course, notably increased the lateral strength of the models. In 1992, eight full-size models of a one-room single-story building were tested on a shake table (PUCP/CIID 1995).
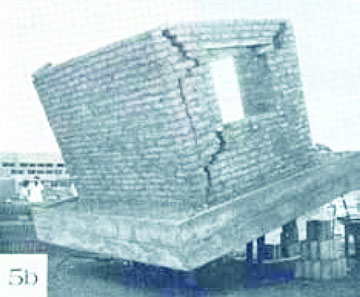
|
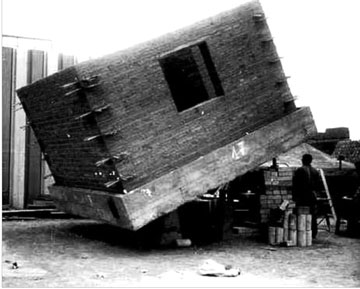
|
Figure 4.2 Seismic performance of an unreinforced and a strength- ened adobe building: a) Unreinforced specimen, and b) Specimen with cane reinforcement (Blondet et al. 2002) |
The test results showed that horizontal and vertical cane reinforcement, combined with a solid ring beam, can prevent wall separation at the corners, even in the case of a severe earthquake.Structural integrity can be maintained even after the walls suffer significant damage. The reinforcement proved to be very effective in preventing building collapse. Many adobe houses reinforced with cane have been built in Peru and El Salvador. The construction of a house in El Salvador is shown in Figure 4.3a (Dowling 2002). Figure 4.3b shows the vertical and horizontal cane mesh being tied together. In some cases in El Salvador, the crushed cane was replaced with barbed wire as horizontal reinforcement, as shown in Figure 4.3c (Dowling 2002). Figure 4.3d shows a finished adobe house reinforced with internal cane mesh and pilasters in Peru.
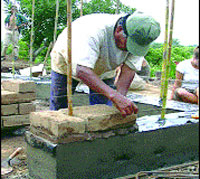
|
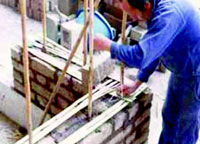
|
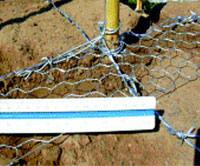
|
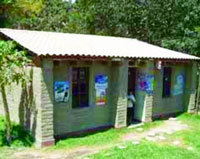
|
Figure 4.3 Construction with cane reinforcement in Peru and El Salvador: a) Placement of cane reinforcement, b) Vertical and horizontal reinforcement, c) Barbed wire as horizontal reinforcement, and d) House reinforced with cane and pilasters |
In addition to a ring beam and cane reinforcement, the use of truss like timber ties between the lintel and ring beam proved to be effective, based on the seismic simulation (shake table) tests performed at the PUCP (Blondet et al. 2002). The performance of an unreinforced adobe building model and a model with vertical and horizontal cane reinforcement, a ring beam and truss like ties is illustrated in Figure 4.4.
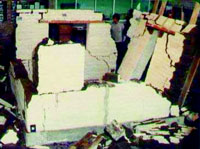
|
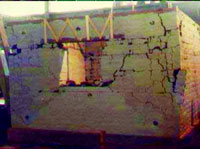
|
Figure 4.4 Adobe building models after shake table tests: a) Unreinforced adobe building, and b) Strengthened adobe building |
It is very difficult to find enough cane for massive reconstruction programs after a strong earthquake. As a solution for this problem, the PUCP tested industrial materials (which are available in large quantities) as internal reinforcement for adobe buildings. An adobe model reinforced with an internal mesh made of vertical PVC tubes and horizontal plastic mesh subjected to a cyclical lateral load showed adequate seismic behavior and proved the efficiency of this technique (Blondet et al. 2005, Madueno 2005).
External cane and rope mesh
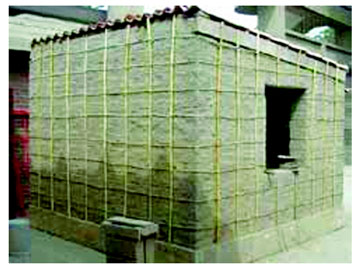
|
Figure 4.5 An adobe building model with external vertical cane and horizontal rope reinforcement |
An external reinforcement system consisting of vertical cane tied with horizontal ropes forming an ap- proximately 450 mm square mesh can be used to wrap adobe walls, as shown in Figure 4.5. An adobe building model with this reinforcement system was tested on the PUCP shake table (Torrealva 2005) and even though severe cracking occurred, this reinforcement scheme successfully prevented collapse system has been developed and tested at the This seismic simulator of the University of Technology, Australia (Dowling et al. 2005). U-shaped adobe wall panels were reinforced with internal chicken wire mesh placed horizontally every three courses. Polypropylene strings were woven through the mesh and its ends were left free, perpendicular to the wall. After the wall was finished, the strings were used to attach vertical bamboo reinforcements placed externally on both sides of the wall. An upper timber ring beam completes the system. The vertical bamboo reinforcements were secured to the ring beam, thus ensuring the complete support of the wall, as shown in Figure 4.7. A Variation of this system uses horizontal instead of vertical bamboo externally on the outside of the wall(Figure 4.7b). Testing has shown that this reinforcement system significantly improves the seismic behavior of wall panels, preventing their collapse.
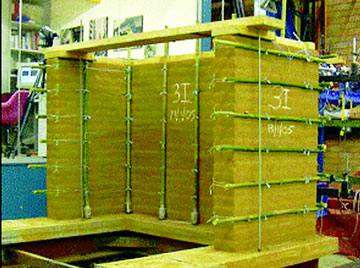
|
Figure 4.6 Adobe building models with external bamboo |
Welded wire mesh
The use of external welded wire mesh has been studied at PUCP as a reinforcement system that could be applied both to new and existing earthen construction (San Bartolome et al. 2008, Zegarra et al. 1997a, 1997b, 2001). Welded mesh reinforcement consists of 1 mm diameter wires at 20 mm spacing nailed with metal bottle caps to the adobe walls, as shown in Figure 4.7a (Zegarra et al. 1997b). The mesh is placed in horizontal and vertical strips, simulating beams and columns, and it is covered with a 20 mm thick cement and sand mortar. Shake table tests were performed on U-shaped walls with and without reinforcement (Zegarra et al. 1997a). The walls were subjected to the same simulated earthquake shaking. An unreinforced model collapsed, while the reinforced model suffered damage, but did not collapse, as shown in Figure 4.7b. For more information related to this technique refer to Quiun (**YEAR**).
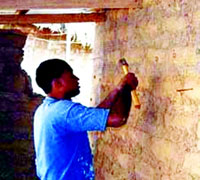
|
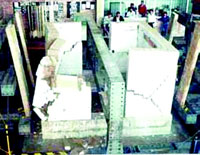
|
Figure 4.7 Welded wire mesh reinforcement: a) Mesh installation, and b) Shake table testing of U-shaped walls |
A pilot construction program in the area affected by the 2001 Arequipa, Peru earthquake (Mw = 8.4) provided valuable information on the seismic performance of buildings strengthened using this technique. Adobe houses that were built by the program with external welded mesh reinforcement covered by cement and sand mortar withstood the seismic event without any damage. Similar houses without rein- forcement collapsed or were severely damaged. Figure 4.8a shows a house in Moquegua, Peru with external welded mesh reinforcement which remained undamaged (front), whereas a house without rein- forcement (shown from the back in the same photo) was severely damaged in the earthquake (Zegarra et al. 2001). This seismic reinforcement scheme also proved to be effective during the 2007 Pisco, Peru earthquake (Mw = 8.0). Houses reinforced with welded wire mesh in Ica showed excellent seismic performance and did not suffer any damage (San Bartolome et al. 2008), as shown in Figure 4.8b. The collapsed adobe wall adjacent to the reinforced house (to the far right in the same photo) was built by the owner without any seismic reinforcement.
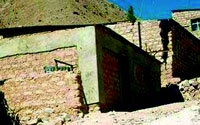
|
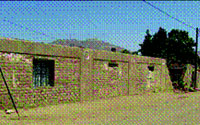
|
Figure 4.8 Reinforced adobe houses with welded wire mesh that withstood earthquakes in Peru: a) Reinforced adobe house in Moquegua, and b) Reinforced adobe house in Ica |
Polymer mesh
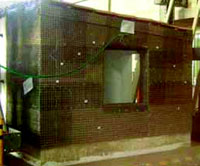 |
A PUCP research team developed a seismic reinforcing technology for adobe houses using externally applied polymer mesh (geomesh), which is commonly used for geotechnical applications (Blondet et al. 2006, Blondet et al. 2008, USAID 2001, 2001a). The mesh is attached to adobe walls by plastic or nylon strings placed during construction. This reinforcing scheme demonstrated excellent seismic response during high intensity shake table tests (roughly equivalent to MM 7) on a full-scale adobe building model, as shown in Figure 4.9. The geomesh reinforcement increased the stiffness, strength and deformation capacity of the adobe walls. Total building collapse was prevented due to the confinement provided by the mesh. The researchers found that it is possible for the walls to disintegrate into large blocks during severe ground shaking, however the mesh prevents the walls from falling apart, and collapse can be avoided (Blondet et al. 2006).
Construction booklets for the geomesh reinforcing scheme were developed by PUCP and CARE Peru after the 2007 Pisco earthquake. CARE Peru is an NGO experienced in developing training programs in rural areas. These booklets explain how to build a safe adobe house reinforced with geomesh for coastal (arid) areas and highland areas (Vargas et al. 2007a, 2007b).
The construction technique indicated in the manuals was revalidated with shake table tests at PUCP. Two adobe models were subjected to seismic motion to prove the efficiency of the geomesh technique (Bossio 2010). Figure 4.10 shows an adobe model reinforced with geomesh and covered with mud plaster during the shake table test.
|
Figure 4.9 Reinforced adobe house with geomesh duing a shake table test at the PUCP.
|
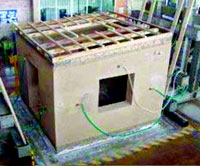 |
Figure 4.10 Adobe model reinforced with geomesh and covered with mud plaster (source: Bossio 2010) |
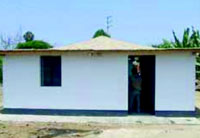
|
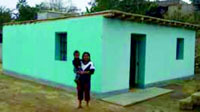
|
Figure 4.11 Reinforced adobe houses in Chincha, Peru built after the 2007 Pisco earthquake: a) A model house from PUCP-CARE's booklet, and b) Reinforced adobe house built by CARE |
After the 2007 Pisco earthquake, PUCP and CARE Peru implemented a training program on safe ado- be construction (Blondet et al. 2008). Nine adobe houses were constructed by the previously trained owners. More than 800 community members participated in the training and adopted the new technology. Figure 4.11a shows a model house featured in the PUCP-CARE booklet. Furthermore, currently (August 2010) more than 1000 adobe houses have been built using the geomesh technology by NGOs and International Cooperation Agencies in the areas affected by the Pisco, Peru earthquake. Figure 4.11b shows a reinforced adobe house built by CARE Peru.
Used car tire straps
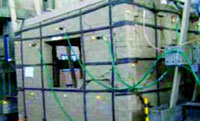 |
This scheme uses circumferentially cut straps from the treads of used car tires for tension reinforcement. Continuous straps pass through holes drilled in the adobe walls to wrap them horizontally every 600 mm and vertically every 1.2 m approximately. This reinforcement enhances the in-plane and out- of-plane resistance of adobe walls to seismic effects (Figure 4.12). Vertical straps pass under the foundations, rise up the walls, and are nailed to the timber wall top plate. The scheme was developed at Victoria University in New Zealand and shake table testing was performed at PUCP. Results showed that the tire strap |
Figure 4.12 Shake table testing of an adobe building model reinforced with tire straps |
reinforcement system prevented building collapse, even during simulated ground shaking of high intensity (Charleson and Blondet 2010, under review). A construction manual "………" that describes how the system can be applied to new and existing houses is available from the World Housing Encyclopedia website (***).
Polypropylene (PP) bands
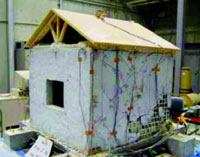 |
PP-band retrofitting is a simple and low-cost method that consists of confining all adobe walls with a mesh of PP-bands. PP-bands are an inexpensive, durable, strong, and widely available material, commonly used for packing. Shake table tests were performed to verify the efficiency of this technique. Figure 4.13 shows a full-scale adobe model reinforced with PP- bands after a shake table test (Meguro 2008). The scheme was developed in Japan. |
Figure 4.13 Full-scale adobe model reinforced with PP-bands after a shake table test |
Integral masonry system
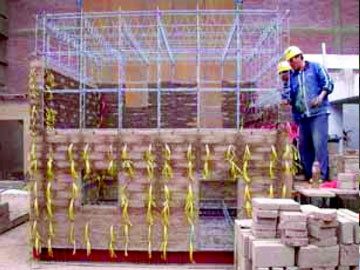
|
Figure 4.14 Construction of a building model using the integral msonry system
|
This system consists of prefabricated electro-welded galvanized wire truss reinforcements which intersect in the three spatial directions, as shown in Figure 4.14. A half-scale model of a two-story building was built using the integral masonry system and was test- ed as a part of a joint research project between the Polytechnic University of Madrid and PUCP. Adobe block infill was used to provide enclosure and ensure sufficient stiffness (Adell et al. 2010). The model withstood seismic simulation without significant cracking.
Table 1 Reinforcement Schemes for Adobe Walls: A Comparison
Wall reinforcement scheme
|
Type of building
|
Construction complexity
|
Cost
|
Seismic safety
|
New
|
Existing
|
Simple
|
Moderate
|
Complex
|
Low
|
Moderate
|
High
|
Low
|
Moderate
|
High
|
Internal cane reinforcement
|
X
|
|
|
X
|
|
X
|
|
|
|
|
X
|
External cane and rope mesh
|
X
|
X
|
X
|
|
|
X
|
|
|
|
|
X
|
External bamboo and internal wire mesh
|
X
|
X
|
X
|
|
|
X
|
|
|
|
|
X
|
Welded wire Mesh
|
X
|
X
|
X
|
|
|
|
X
|
|
|
X
|
|
Polymer Mesh
|
X
|
X
|
X
|
|
|
|
X
|
|
|
|
X
|
Used car tire straps
|
X
|
X
|
|
X
|
|
X
|
|
|
|
X
|
|
Polypropylene band
|
X
|
X
|
|
X
|
|
X
|
|
|
|
|
X
|
Integral masonry system
|
X
|
|
|
|
X
|
|
|
X
|
|
|
X
|
Table 1 presents a comparison of various wall reinforcement schemes. The first parameter refers to the type of application (new or existing houses). The second parameter is related to the complexity of construction, ranging from simple to complex. The third parameter classifies each reinforcement system according to cost, ranging from low to high. The last parameter ranks the reinforcement systems ac- cording to the seismic safety they provide. Note that the objective of providing seismic reinforcement in a building is to protect the lives of the building occupants, that is, life safety.
Buttresses and Pilasters
Buttresses and pilasters are provided at critical loca- tions to increase the overall stability and strength of a building. Buttresses act as restraints, preventing the inward or outward collapse of walls. Buttresses and pilasters must be used in addition to wall reinforce- ment to ensure adequate seismic safety. This section is based on the recommendations by IAEE (1986) and Dowling (2004b).
The critical locations are:
- Corners, where buttresses take the form of over- lapped (crossed over) walls
- Intermediate locations in long walls, where pilas- ters are constructed as bracing walls in the transverse direction and are integrated into the wall structure.
Buttresses and pilasters were used to improve the seismic resistance of adobe construction in El Salvador as part of a grass root education and rebuilding effort following the 2001 earthquakes (Dowling 2004a). Furthermore, buttresses and pilasters were effective in preventing the collapse of adobe buildings in Santiago during the 2010 Maule, Chile earthquake.
Seismic Protection of Historic Adobe Buildings
Historic adobe buildings, regardless of their important architectural or cultural value, are prone to earth- quake damage like other adobe structures. It is impor- tant to ensure earthquake life safety while performing minimal intervention on their original fabric.
As part of the Getty Seismic Adobe Project (GSAP) sponsored by the Getty Conservation Institute, nine small-scale (1:5) model buildings were tested on the shake table at the John A. Blume Earthquake Center at Stanford University in Palo Alto, California (Tolles et al. 2000). Two large-scale (1:2) model buildings were tested during the final phase of the GSAP program at the Institute of Earthquake Engineering and Engineering Seismology (IZIIS), Republic of Macedonia. The most effective retrofitting provisions studied in the project are briefly described below.
- Nylon straps made of 3 mm wide, flexible, woven nylon were placed horizontally or vertically, forming a loop, either around the entire building or around an individual wall. The straps were passed through small holes in the wall and the two ends were knotted together. Vertical straps were most effective in reducing the risk of out-of-plane wall collapse.
- Vertical center core elements were drilled through the adobe walls. These elements consisted of 3.0 mm or 4.8 mm diameter steel rods anchored with an epoxy grout. Prior to drilling the rods into the wall, each end was flattened into a V-shaped form. These elements were found to be particularly effective in increasing both the in-plane and out-of-plane wall resistance.
- Wood bond beams were anchored to the walls with coarsely threaded screws or partial wood diaphragms. Cross-ties made out of nylon cord were installed to reduce the differential displacement across the cracks and to provide a through-wall connection.
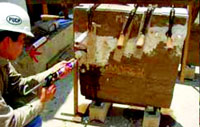
|
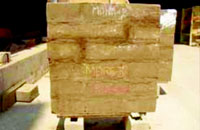
|
Figure 5.2 Grout injection procedure: a) Crack injection, and b) Repaired wall |
Earthquake induced cracks are a serious problem for historic adobe buildings. These cracks cause a decrease in the strength and stiffness of adobe walls, thus increasing the risk of collapse in future earthquakes (Blondet et al. 2007). A PUCP research team developed a technique to repair the cracks in historic adobe buildings and restore the original strength of the structure by injecting mud-based grout into the wall. The grout is pumped into the cracks through 3 mm diameter holes made of plas- tic tubes, as shown in Figure 5.2. Before grouting, the cracked surfaces need to be sealed with gypsum and injected with water.
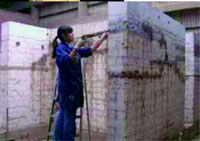
|
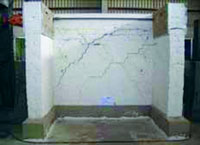
|
Figure 5.3 Repairing seismic cracks on adobe buildings at PUCP: a) Crack injection on adobe module, and b) Repaired module being tested |
Grout compositions considered in this study were mud-based, either plain, or stabilized with cement, lime, and/or gypsum. Many indirect tension tests were performed using adobe "sandwiches" (two adobe blocks joined by mud mortar) to establish which grouts provided an adequate bond between the mortar and the adobe blocks. The thickness of the mortar joints ranged from 2 to 10 mm. The soil used for the mortar mix was sifted to remove particles larger than 2 mm. Some mortar mixes were stabilized with cement and lime (5%, 7%, and 10% ratio), and gypsum (5%, 10%, and 20% ratio). The results showed that sandwiches with a thinner mortar joint result in an increase in adobe masonry strength.
Diagonal compression tests were performed on re- paired adobe panels with the most efficient grouts. The results showed that grouting is an effective method for repairing cracks in adobe walls when crack widths are in the range of 3 mm to 5 mm. Mud-based grout using soil sifted with a #10 (2 mm) sieve and moisture content ranging from 30% to 40% was carefully injected in the walls. Tests showed that the wall was restored to its original strength. The grout mix consisting of soil stabilized with gypsum (20% to 30% ratio) also proved to be effective, how- ever the injection process was more complex.
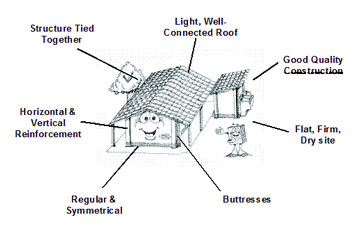 |
Another PUCP research team studied the feasibility of grouting seismic cracks of less than 3 mm wide. The conclusion was that to ensure efficient grout- ing, it is necessary to make thin cracks wider, so the grout can be applied totally. This technique was used to repair adobe modules previously subjected to a cyclical lateral load (thus, with "real" seismic cracks). The grout used to repair the opened cracks on the adobe modules was a mixture of straw and soil sifted by a #10 sieve (with a moisture content of around 35%). The tests on the repaired adobe modules concluded again that grouting is an efficient way to restore the original strength of adobe walls with minimal intervention on their original fabric. Figure 5.3 shows a crack injection and the cyclical lateral load test. The main recommendations for the improved seismic performance of adobe construction are summarized below
References
Adell, J., G-Santos, A., Orta, B., Bustamante, R., Peña, J., Blondet, M., Ginocchio, F., and Villa Garcia, G., 2010. Earthquake - Integral Masonry System tested in Lima: Buildings behaviors of Adobe & Hollow Brick, Pro- ceedings, 8th International Masonry Confer- ence, Dresden, Germany.
AIS, 2004. Manual para la Rehabilitación de Viviendas Construidas en Adobe y Tapia Pisada, Asociacion Colombiana de Ingeneria Sismica, http://www.preventionweb.net/english/professional/trainings-events/edu- materials/v.php?id=5208.
Astroza M., Cabezas, F. M., Moroni, M. O. Y., Mas- sone, L. S., Ruiz, S. T., Parra, E., Cordero, F. O., Mottadelli, A. S., 2010. Intensidades Sismicas en el Area de Danos del Terremoto del 27 de Febrero de 2010, Departamento de Ingeniería Civil, Facultad de Ciencias Físicas y Matemáti- cas, Universidad de Chile, Santiago, Chile.
Bariola, J., Blondet, M., Torrealva, D., and Vargas J., 1986. Comportamiento Dinámico de Viviendas de Adobe (Dynamic Behavior of Adobe Dwellings), VI Congreso Nacional de Ingeniería Civil, Cajamarca, Perú.
Blondet, M., Torrelva, D., and Villa Garcia, G., 2002. Adobe in Peru: Tradition, Research and Future, Modern Earth Building 2002
- International Conference and Fair, Berlin, Germany.
Blondet, M., and Villa Garcia, G., 2004. Adobe Construction, World Housing Encyclopedia - Summary Publication, Earthquake Engineering Research Institute, Oakland, CA.
Blondet, M., Torrealva, D., Villa Garcia, G., Ginocchio, F., and Madueño, I., 2005. Using Industrial Materials for the Construction of Safe Adobe Houses in Seismic Areas, Earth- Build2005 Conference, University of Technol- ogy, Sydney, Australia.
Blondet., M, Vargas, J., Ginocchio, F., Morales, K., and Iwaki, C., 2007. Estudio Preliminar del
Uso de Grouts de Barro para Reparar Fisuras Estructurales en Muros Históricos de Adobe, AdobeUSA 2007 - NNMC and Adobe Asso- ciation of the Southwest, El Rito, NM.
Blondet, M., Vargas, J., Patron, P., Stanojevich M., and Rubiños, A., 2008. A Human develop- ment approach for the construction of safe and healthy adobe houses in seismic areas,
14th World Conference on Earthquake Engi- neering, Beijing, China.
Blondet, M. (editor), 2010. Construcción de viviendas sismorresistentes y saludables de adobe reforzado con geomallas, primera edición, Catholic University of Peru, Editorial Fund, Lima, Peru.
Bossio, S., 2010. Evaluación del comportamiento sísmico e influencia de la dirección del mov- imiento en módulos de adobe reforzado con geomalla, Catholic University of Peru, Lima, Peru.
CENAPRED, 2000. Métodos de Refuerzo para la Vivienda Rural de Autoconstrucción (Rein- forcement Methods for Self construction of Rural Housing), México City, Mexico.
Charleson, A., and Blondet, M., 2010. Seismic Reinforcement for Adobe Houses with Straps from Used Car Tires, Earthquake Spectra, under review.
Coburn, A., Hughes, R., Pomonis, A., and Spence, R., 1995. Technical Principles of Building for Safety, Intermediate Technology Publications, London, England.
CTAR/COPASA, GTZ, PUCP, SENCICO, 2002.
Terremoto? ¡Mi casa sí resiste! (Earthquake? My house withstands!), Arequipa, Peru.
De Sensi, B., 2003. Terracruda, La Diffusione Dell'architettura Di Terra (Soil, Dissemina- tion of Earth Architecture), www.terracruda.com/architetturadiffusione.htm.
Dowling, D. M., 2002. Improved Adobe in El Sal-vador. Power Point presentation to Earthquake Engineering Research Institute, Oakland, CA.
Dowling, D, 2004a. Adobe Housing Reconstruc- tion after the 2001 El Salvador earthquakes, Learning From Earthquakes Series, Volume V, Earthquake Engineering Research Institute, Oakland, CA.
Dowling, D. M., 2004b. Adobe housing in El Sal- vador: Earthquake performance and seismic improvement, in Natural Hazards in El Sal- vador (W. I. Rose, J. J. Bommer, D. L. Lopez, M. J. Carr, and J. J. Major, eds.), Geological Society of America, Boulder, CO, 281-300.
Dowling D, Samali B and Li J. 2005. An Improved Means of Reinforcing Adobe Walls: External Vertical Reinforcement, SismoAdobe 2005, Lima, Peru.
EERI, 2004. Preliminary Observations on the
Bam, Iran, Earthquake of December 26, 2003, Earthquake Engineering Research Insti- tute Newsletter, April 2004, Oakland, CA.
EERI, 2008. The Wenchuan, Sichuan Province, China, Earthquake of May 12, 2008, Earth- quake Engineering Research Institute Newslet- ter, October 2008, Oakland CA.
Equipo Maíz, 2001. La Casa de Adobe Sismorre- sistente (Earthquake-Resistant Adobe House), Equipo Maíz Association, El Salvador, 91pp. Houben, H., and Guillaud, H., 1994. Earth Con- struction - A Comprehensive Guide, ITDG Publishing, London, England. IAEE, 1986. Guidelines for Earthquake-Resistant
Non-Engineered Construction, International Association for Earthquake Engineering, Tokyo, Japan, http://www.nicee.org/NICEE/Manuals/iaee/iaeemanual_1.html
INEI, 2007. XI Population and VI Housing Census (in Spanish), Statistics and Informatics National Census, http://iinei.inei.gob.pe/iinei/RedatamCpv2007.asp?ori=C
INEI, 2007. Census of Affected Areas by the August 15, 2007 Earthquake (in Spanish), Statistics and Informatics National Census, http://www1.inei.gob.pe/sismo2007/Resulta-dos1.asp?resultado=6
Madueño, I., 2005. Reforzamiento de Construc- ciones de Adobe con Elementos Producidos Industrialmente: Estudio Preliminar, Catho- lic University of Peru, Lima, Peru.
Meguro, K., 2008. Technological and Social Ap- proaches to Achieve Earthquake Safer Non- engineered Houses, 14th World Conference on Earthquake Engineering, Beijing, China
Neves, C., Faria, O., Rotondaro, R., Cevallos, P., and Hoffmann, M., 2009. Selección de suelos y métodos de control en la construcción con tierra - prácticas de campo, Available on http://www.redproterra.org.
Office of the Registrar General & Census Commis- sioner, India, 2001. Census of Indiahttp://www.censusindia.gov.in/Census_Data_2001/Census_data_finder/Census_Data_Finder.aspx/
Papanikolaou, A. and Taucer, F., 2004. Review of Non-Engineered Houses in Latin America with Reference to Building Practices and Self-Construction Projects, European Com- mission, Joint Research Centre, Institute for the Protection and Security of the Citizen
European Laboratory for Structural Assessment (ELSA), Ispra, Italy, http://elsa.jrc.ec.europa.eu/showdoc.php?object_id=26.
PUCP/CIID, 1995. Nuevas Casas Resistentes De Adobe (New Earthquake-Resistant Adobe Houses), Catholic University of Peru, Centro Internacional de Investigacion Para el Desar- rolo (CIID), Lima, Peru.
Rael, R., 2009. Earth Architecture, Princeton Architectural Press, New York, NY.
RESESCO, 1997. Reglamento Para la Seguridad Estructural de las Construcciones: Folleto Complementario Adobe (Regulations for the Structural Safety of Construction: Comple- mentary Brochure Adobe), Asociación Salva- doreña de Ingenieros y Arquitectos, Ministerio de Obras Públicas, El Salvador, 36 pp.
San Bartolome, A., Quiun, D., and Zegarra, L., 2008. Performance of Reinforced Adobe Houses in Pisco, Peru Earthquake, 14th World Conference on Earthquake Engineering, Beijing, China.
Tolles, E. L., Kimbro, E. E., Webster, F. A., and Ginell, W. S., 2000. Seismic Stabilization of Historic Adobe Structures - Final Report of the Getty Seismic Adobe Project, GCI Scien- tific Program Reports, The Getty Conservation Institute, Los Angeles, CA, http://www.getty.edu/conservation/resources/seismicstabilization. pdf.
Tolles, E.L., Kimbro, E. E., and Ginell, W. S., 2002. Planning and Engineering Guidelines for the Seismic Stabilization of Historic Adobe Structures, GCI Scientific Program Reports, The Getty Conservation Institute, Los Angeles, CA.
Torrealva, D. and Acero, J., 2005. Reinforcing Adobe Buildings with Exterior Compatible Mesh. The Final Solution Against the Seis- mic Vulnerability? SismoAdobe 2005, Lima, Peru.
USAID, 2001. Peru Earthquake Fact Sheet #6, FY 2001, http://www.reliefweb.int/w/rwb.nsf/vLND.
USAID, 2001a. El Salvador Earthquakes Janu- ary 13 and February 13, 2001, Consolidated Data by Department, http://www.releifweb.in/w/map.nsf/wByCLatest.
Vargas, J., Bariola, J., and Blondet, M., 1984. Seis- mic Strength of Adobe Masonry, Summary of a research project sponsored by the US Agency for International Development (AID), Lima, Peru.
Vargas, J. and Blondet, M., 2005. 35 years of Sis- moAdobe research at the Catholic University of Peru (in Spanish), SismoAdobe 2005: Inter- national Conference on Architecture, Conser- vation and Construction of Adobe Buildings in Seismic Areas, Catholic University of Peru, Lima, Peru.
Vargas, J., Torrealva, D., and Blondet, M., 2007a. Building hygienic and Earthquake-Resistant Adobe Houses Using Geomesh Reinforce- ment: For Arid Zones (in Spanish and Eng- lish), Catholic University of Peru, Editorial Fund, Lima, Peru, http://www.world-housing.net.
Vargas, J., Torrealva, D., and Blondet, M., 2007b. Building Hygienic and Earthquake-Resistant Adobe Houses Using Geomesh Reinforce- ment: For Highland Zones (in Spanish), Catholic University of Peru, Editorial Fund, Lima, Peru, http://www.world-housing.net
Zegarra, L., Quiun, D., San Bartolome, A., and Gisecke, A., 1997a. Reforzamiento de Vivien- das de Adobe Existentes. 1era Parte: Ensayos Sísmicos de Muros 'U' (Reinforcement of Ex- isting Adobe Houses. 1st Part: Seismic Tests of "U" Shaped Walls), Summary of a research project sponsored by CERESIS-GTZ-PUCP, XI CONIC, Trujillo, Peru.
Zegarra, L., Quiun, D., San Bartolome, A., and Gisecke, A., 1997b. Reforzamiento de Vivien- das de Adobe Existentes. 2da Parte: Ensayos Sísmicos de Módulos (Reinforcement of Ex- isting Adobe Houses. 2nd Part: Seismic Tests of Housing Modules), Summary of a research project sponsored by CERESIS-GTZ-PUCP, XI CONIC, Trujillo, Perú
Zegarra, L., Quiun, D., San Bartolome, A., and Gisecke, A., 2001. Comportamiento ante el Terremoto del 23-06-2001 de las Viviendas de Adobe Reforzadas en Moquegua, Tacna y Arica (Behavior of Reinforced Adobe Houses in Moquegua, Tacna and Arica during the 23-06-2001 Earthquake), XIII CONIC, Puno, Peru.
Relevant World Housing Encyclopedia Reports
Kumar, A., 2002. Rural Mud House with Pitched Roof, Report 23 (India).
Lang, D., Holliday, L., and Flores, O., 2007. Ado- be Brick Houses, Report 144 (Guatemala).
Loaiza, C., Blondet, M., and Ottazzi, G., 2002. Adobe House, Report 52 (Peru).
Lopez, M., Bommer, J., and Benavidez, G., 2002. Adobe House, Report 14 (El Salvador).
Mehrain, M. and Naeim, F., 2004. Adobe House, Report 104 (Iran).
Quiun, D., 2009. Reinforced Adobe, Report 107 (Peru).
Rodriguez, V., Yacante, M., and Reiloba, S., 2002. Traditional Adobe House with Reinforcement, Report 2 (Argentina).